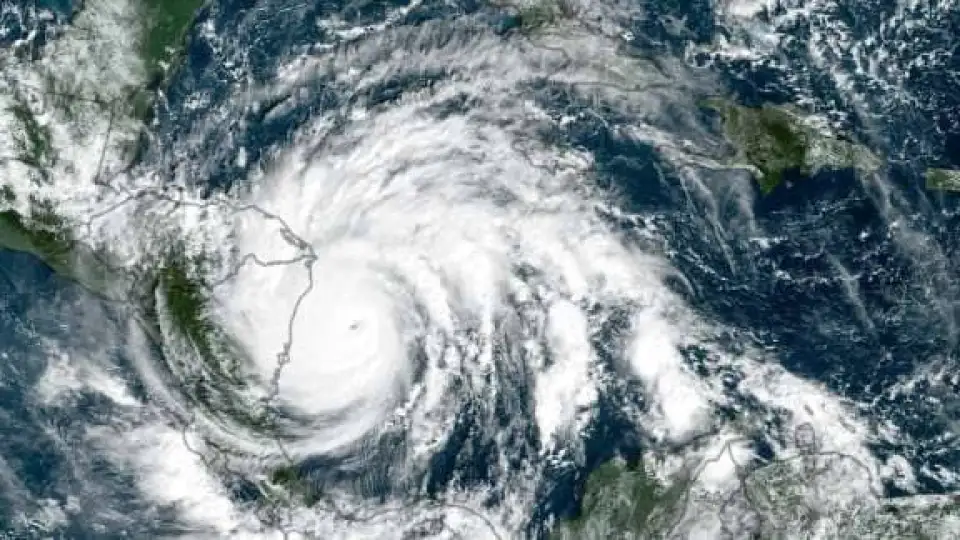
In 2018, the Intergovernmental Panel on Climate Change (IPCC) published a special report on the impacts of a 1.5°C (2.7°F) increase in global warming over preindustrial levels. According to this report, global greenhouse gas (GHG) emissions must be reduced to at least 45% and 100% below 2010 levels by 2030 and 2050, respectively, if we are to avert catastrophic climate change.1 With organizations, governments, and individuals increasingly focused on reducing emissions, much attention has been paid to improving the energy efficiency of the built environment.
Buildings play an important role in addressing climate change. Globally, building operations account for 28% of annual GHG emissions. Strategies such as improving airtightness or increasing the thermal performance of a building’s envelope can therefore contribute significantly to reducing building emissions, especially if those buildings are heated using fossil fuels, or powered by an energy grid reliant on fossil fuels.
As buildings become ever more efficient and utility grids decarbonize, the role played by operational emissions will be surpassed by the role played by emissions embodied in the buildings themselves. In construction, embodied emissions are defined as the GHG emissions released during the extraction, transportation, manufacturing, construction, demolition, and disposal of a given material or product. These GHG emissions are primarily released prior to and during the building’s construction, with a fraction of the emissions released at the end of a product’s service life.
As it stands now, the embodied emissions of building materials, including the emissions released during construction, account for 11% of annual GHG emissions.3 The production of concrete alone is responsible for over 9% of annual emissions.4 Due to the long atmospheric lifetime of certain greenhouse gases and the feedback loops they generate, the emissions produced within the next decade will have a significantly greater impact on our ability to address climate change within the next 30 years than those produced in subsequent years.
Compounding the problem, future emissions are often reduced at the expense of current emissions; improvements in the energy efficiency of a building are often tied to increased embodied emissions resulting from additional insulation materials, more-complex mechanical systems, or the use of triple-pane rather than double-pane insulated glass units, for example. It is therefore incumbent on architecture, engineering, and construction (AEC) professionals to properly assess and address the entire life cycle impacts of a given building in order to strike the appropriate balance between embodied and operational emissions. Fortunately, this balance is gaining increasing attention, and life cycle analysis calculators are becoming increasingly available, as detailed below.
A good starting point is to look at the emissions profile of the local electricity grid. The EnergyStar Greenhouse Gas Emissions Technical Reference guide provides emissions factors for Canadian and American utility grids.5 If the grid is supplied by a coal, diesel, or gas- fired power plant, operational emissions will probably still play a dominant role in the emissions profile of a building. Similarly, if the client or engineers insist on heating with fossil fuels, the embodied emissions associated with increased energy efficiency beyond code requirements should quickly be offset by the resulting reductions in operational emissions. However, if the local grid has relatively low GHG emissions, or will be transitioning to fossil-fuel-free means of energy production, the calculus rapidly changes.
There is, however, a caveat that is of the utmost importance. Not all building materials are created equal. While some building materials are energy and emissions intensive to produce, and others slightly less so, some building materials actually remove carbon from the atmosphere during their production. An increased use of these materials in a given construction project—an increased use of cellulose or cork for insulation, for example—could not only reduce the embodied carbon of the building, but also reduce operational emissions.
Although the use of plant-based materials is an important first step to reduce embodied emissions, it is just as important to ensure that those materials are sustainably sourced. In a study comparing the embodied emissions of a 12-story tower built using either concrete or timber, the authors found that while sustainably sourced timber from local manufacturers would sequester roughly as much carbon as the use of concrete would emit, the use of timber from non-sustainable forestry sourced at a distance would have an emissions profile approaching that of concrete.6
The use of agricultural residues and waste fibers represents an even more interesting alternative to conventional construction materials. Not only could materials made from these agricultural by-products sequester carbon, but they would also reduce agricultural waste and maximize the useful output of arable land. Agricultural products also have a significantly shorter growing cycle than timber, and given that the agricultural residues and waste fibers are already in continuous production, their use in construction materials offers the potential to rapidly and cost-effectively reduce the GHG emissions of the built environment.
The degree to which plant-based materials sequester carbon remains somewhat contentious. However, there is little disagreement as to whether their integration into the built environment is currently one of the best strategies to reduce the embodied carbon emissions of buildings.
For AEC professionals looking to take a more-sophisticated approach to reducing the life cycle GHG emissions of their projects, a variety of life cycle analysis (LCA) tools are currently available on the market. Some commercially available software, such as Tally or One Click LCA, offer the ability to quickly conduct LCAs directly from BIM software, such as Revit. Others, such as the Athena Impact Estimator for Buildings, offer a free LCA alternative requiring slightly more manual input. While tools like EC3 focus exclusively on the quantification of embodied carbon, as opposed to a complete LCA. In all cases, these tools empower AEC professionals to make informed construction material choices specific to the context of a given project.
For design professionals familiar with the Passivhaus PHPP energy modeling software, I have developed a free and open source Microsoft Excel-based carbon analysis plugin, the PHPP v9.6 Carbon Calculator. The analysis is limited to projects located in Canada and the United States. The tool focuses exclusively on the embodied carbon of insulation materials as compared to the energy performance and resulting GHG emissions, of a given project over a 60-year period. By using this dynamic simulation tool during the design phase of a project, the selection and quantity of insulation materials can be optimized for the project’s specific energy grid to ensure that both embodied and operational GHG emissions are minimized over the entire lifecycle of the building.
As LCA tools become more refined and datasets more robust, AEC professionals will be able to hone their designs to shift the focus from simply lowering embodied carbon emissions to maximizing sequestered carbon, all while minimizing operational GHG emissions. It is both refreshing and empowering to consider that growth in the construction sector could serve as a climate change mitigation strategy when the appropriate material and design choices are made.
Philippe St-Jean is McGill University’s sustainable construction officer.
IPCC. (2018). Global Warming of 1.5°C. An IPCC Special Report on the impacts of global warming of 1.5°C above pre-industrial levels and related global greenhouse gas emission pathways, in the context of strengthening the global response to the threat of climate change, sustainable development, and efforts to eradicate poverty. Retrieved from https://www.ipcc.ch/site/assets/uploads/2018/10/SR15_SPM_version_stand_alone_LR.pdf
International Energy Agency and the United Nations Environment Programme. (2018). 2018 Global Status Report: Towards a zero‐emission, efficient and resilient buildings and construction sector. Retrieved from https://wedocs.unep.org/bitstream/handle/20.500.11822/27140/Global_Status_2018.pdf?sequence=1&isAllowed=y
P. J. Monteiro, S. A. Miller, and A. Horvath, “Towards Sustainable Concrete,” Nature Materials 16, no.7 (2017): 698.
U.S. Environmental Protection Agency. (2019). Greenhouse Gas Emissions. Retrieved from https://portfoliomanager.energystar.gov/pdf/reference/Emissions.pdf
B. King, The New Carbon Architecture: Building to Cool the Climate (Gabriola Island: New Society Publishers, 2017).