By Jay Fox
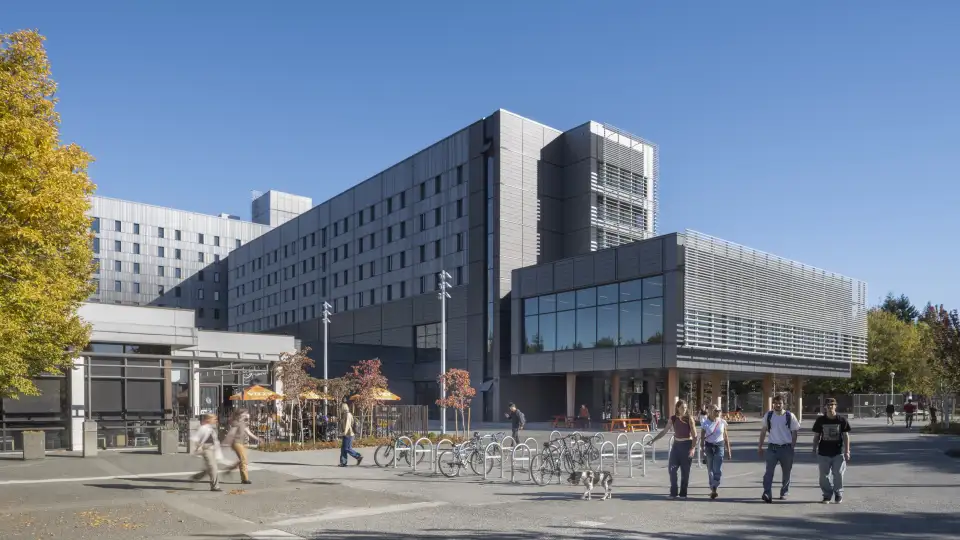
Tips for Designing Commercial Kitchens in Passive House Buildings
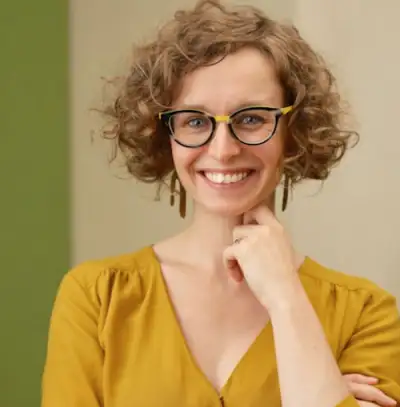
Commercial kitchens have long been considered one of the most daunting challenges in Passive House construction. They are incredibly energy intensive and the use of systems to recover energy can be complicated due to a host of factors unique to large-scale kitchens—most notably, the increased presence of grease and excess moisture that arises when staff is tasked with feeding thousands of people each day. As a result, commercial kitchens continue to be a rarity in passive buildings.
Though they are uncommon, especially in North America, two recently completed projects in Canada—one at the University of Toronto Scarborough (UTSC), the other at the University of Victoria (UVic) in British Columbia—show that it is possible to have a commercial kitchen in a Passive House building. Surprisingly, innovative mechanical systems are only one piece of the puzzle.
According to Marine Sanchez—an engineer and Passive House Specialist with RDH Building Science who was an integral part of both projects—achieving Passive House levels of performance is more about innovative design, teamwork, and thoughtful planning. These are lessons that can be incorporated into any project so long as there is stakeholder buy-in from the very beginning.
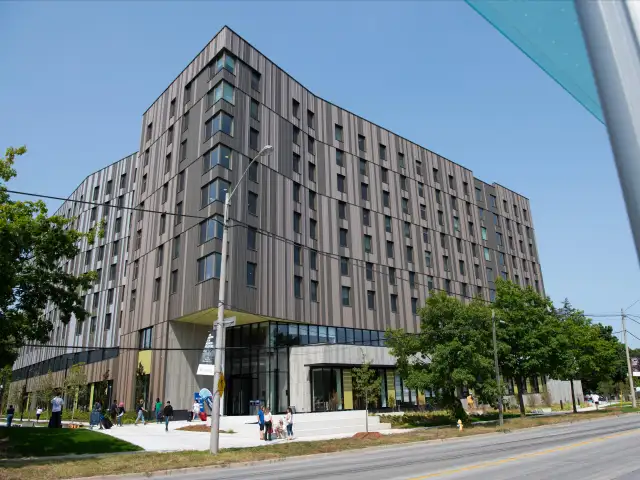
Harmony Commons at a Glance
Gross floor area: 265,000 ft2 (24,620 m2)
Treated floor area: 202,400 ft2 (18,800 m2)
Architects (compliance & client side): CORE Architects Inc. and Handel Architects LLP
Architect (Design-Build team): Arcadis (previously IBI Group)
Passive House Consulting (Design-Build team): RDH Building Science
Passive House Certification: Passive House Institute
The two projects in question are both student residences, though they are quite different. UTSC’s Harmony Commons is a single building that houses almost 750 students and has been responsible for providing over 2,000 meals per day since opening in the fall of 2023. It is currently the largest certified Passive House building in Canada. UVic’s Passive House complex consists of two buildings—Čeqʷəŋín ʔéʔləŋ (Cheko’nien House) and Sŋéqə ʔéʔləŋ (Sngequ House, shown above)—and opened in fall of 2022 and fall of 2023, respectively. The two buildings contain a total of 782 beds, but only Cheko’nien House has been outfitted with a commercial kitchen (to maximize the efficiency coming with scale), which amazingly dishes out around over 9,000 meals each day. That’s a lot of poutine!
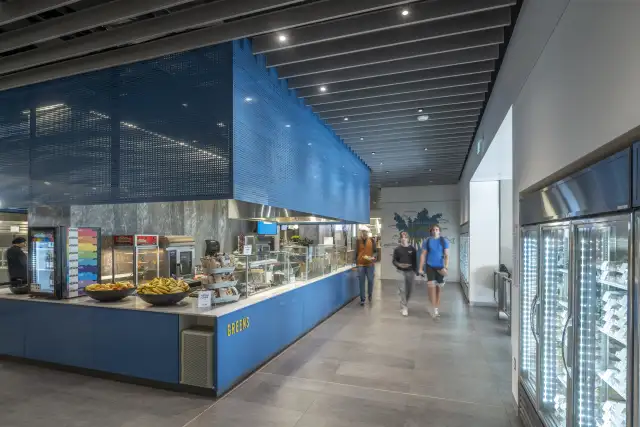
University of Victoria Complex at a Glance
Combined gross floor area of 330,000 ft2 (30,800 m2)
Sngequ House is 11 stories, with 115,500 ft2 (10,750 m2) of treated floor area
Cheko’nien House is 8 stories, with 123,500 ft2 (11,450 m2) of treated floor area
Architects: Perkins&Will
Passive House Consulting: RDH Building Science
Final PHI certification for Cheko’nien House awarded in 2024
Certification for Sngequ House is pending
UVic photography by Michael Elkan
Passive House Rules
Though Sanchez can and will speak at exceptional length about her experience on these two projects, which were truly massive, the focus of this article will be an overview of the strategies that she and Roberto Iannetti, then of the Passive House Institute, developed and employed to bring down energy demand in the kitchen. (For a deeper dive, it is recommended that you check out their presentation from the Passive House Network’s 2021 Passive House for All conference). However, before describing these strategies, there are two interrelated points that are commonly brought up when discussing high-performance construction on any scale, and they are worth repeating.
First, collaboration is key. Passive House design requires a holistic approach to building and this approach only works properly when there is extensive and constant communication between all members of the team. Consequently, team members need to be on the same page from the start, and the energy budget management embedded at the core of the design process in a manner that parallels the financial budget of the project. These energy budgets should be thoroughly incorporated into the design process and all team members should keep them top of mind throughout the decision-making process. This includes everyone: the owner, architects, modelers, engineers, and contractors—and in this case the kitchen staff and kitchen consultant. “It’s a team sport,” Sanchez says.
Second, changing course becomes more expensive and complicated as the team moves through the phases of a project. In essence, an ounce of prevention is worth a pound of cure. Teams should spend the extra time making sure everything is figured out and clearly defined during design so plans don’t have to be amended during construction. This is true even with changes to kitchen equipment or the placement of said equipment. Equipment specification should be made early and all kitchen stakeholders, including members of the kitchen staff, should be consulted early on in the design process. Missing doing so will result in a loss of cost and/or performance optimization. To reiterate the previous point, it takes a village to build one of these buildings, so collaboration is key!
“This approach is identical in concept to Passive House and any high-performance, low carbon design in general,” Sanchez notes. “You frontload early design with heavy collaboration; you plan your strategies early, with a holistic view of systems and their impacts across disciplines; and then the rest of the design is turning your strategies from a set performance target into an exact enclosure assembly, architectural detail, mechanical drawing, specification, and/or product procurement.”
Another strategy worth noting is that both Harmony Commons and Cheko’nien House were divided into two zones—the tower portions of the buildings that contain the student residences and the podium portions of the buildings that contain the kitchens and the non-residential spaces within the buildings (e.g., dining halls, classrooms, lobbies). In the residential zones, the team sought space heating demand targets between 2.5-3.2 kBtu/ft2/yr (8-10 kWh/m2/yr). As the building-wide space heating demand target for Passive House is 4.75 kBtu/ft2/yr (≤ 15 kWh/m2/yr), hitting the lower target in the residential zones allowed the team a more forgiving target of 8 kBtu/ft2/yr (25 kWh/m2/yr) in the non-residential zones.
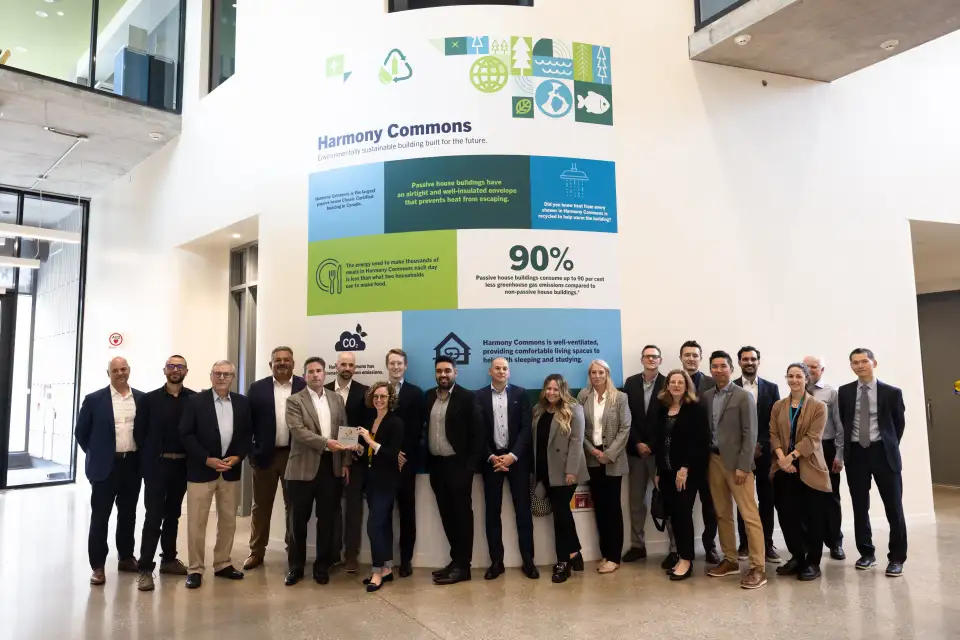
Priorities When Designing a Commercial Kitchen for Passive House
The appliances that cook and preserve our food, as well as the mechanical systems that condition the air in these spaces, are all energy intensive. Commercial kitchens combine that level of intensity with long periods of operation. In the case of the kitchens at UTSC and UVic, they are open for 14 hours per day without breaks between breakfast, lunch, and dinner.
To put this in terms of energy use intensity (EUIs), multifamily Passive House buildings of recent vintage (and without commercial kitchens) can have EUIs between 20-30 kBtu/ft2/yr (63-95 kWh/m2/yr). When performing the energy modeling for the commercial podium space of Cheko’nien House, Sanchez’s calculations placed the commercial podium’s EUI above 150 kBtu/ft2/yr (470 kWh/m2/yr) with all best practice energy saving measures, after achieving a threefold reduction in energy consumption. That is not a typo. In fact, Sanchez was so taken aback by the enormous figure that she did the calculations four times to ensure that these figures were right. These figures also show the extent to which high-performance kitchens can reduce their energy and carbon footprint.
Obviously, the team did not have to start from scratch. PHI had established some of the theoretical guidelines that laid the groundwork for their success, but the team still had to do loads of calculations to assess the impact of each design variable, to recognize the worst offenders, and to come up with practical solutions to make Passive House certification possible. Three key strategies were identified:
1. Right-size all kitchen ventilation systems
2. Use efficient kitchen equipment
3. Recover waste heat
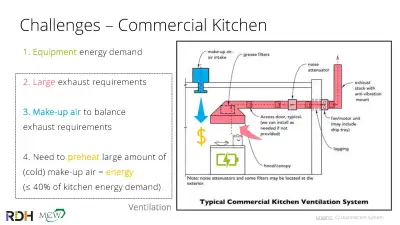
Efficient Equipment & Rightsizing Ventilation Systems
Rightsizing seems like an obvious solution to the problem of wasting energy—it’s right there in the name. Of course, rightsizing is easier said than done because even fully electric kitchens produce enormous amounts of heat, steam, grease, and particulate matter. Large amounts of energy are then needed to remove that stale air and replace it with fresh air. As Figure 1 illustrates, commercial kitchens introduce three interconnected drivers that can frustrate a team’s ability to deliver an optimized ventilation system:
1. Large exhaust requirements
2. Large make-up air requirements to balance exhaust
3. The need to condition the make-up air
Careful planning from the earliest stages of design can minimize the demand for exhaust, thereby reducing the total size of the ventilation system. In some cases, these decisions are matters of design that are cost neutral or add very little to the upfront costs associated with construction (when planned early) but can be justified because they reduce operating costs by improving efficiency. In others, the decisions require upgrading industry-standard systems, which does have some impact on upfront costs.
Design Improvements
Cluster appliances with similar cooking schedules together
Not every appliance in the kitchen is running when the kitchen is open. By separating appliances with different cooking schedules and clustering those with similar schedules, exhaust hoods for the nonoperational appliances can be turned off, instead of running at their minimum exhaust flow rate (flow rate which is generally oversized if only one or two equipment pieces in use rather than the whole food “station”).
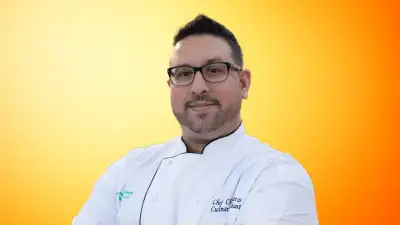
Opt for equipment with less heat production
Ditching gas equipment is a sure-fire way to make kitchen environments more efficient. With gas, approximately 40% of the heat produced by the flame goes towards cooking the food. The remaining 60% is waste heat. With induction cooktops, approximately 84% of the heat that gets generated goes towards cooking the food. Only 16% of the energy is wasted. Though many all-electric kitchen appliances were once difficult to find, Sanchez says that there has been a discernible shift toward electrification in the market and that it is now feasible (and the new normal for a core of food service professionals) to specify all electric kitchens. Chef Chris Galarza, who spoke at Reimagine Buildings: Electrification conference and will soon be a guest on the Passive House Podcast, is one of the food services professionals helping to lead the way.
Applying the principles of Passive House (a continuous, insulated and airtight enclosure) to kitchen appliances will also help to reduce ambient heat, meaning that energy isn’t wasted, and the kitchen is way more comfortable for the staff. For example, appliances with glazing (e.g., ovens, hot food holding cabinets, wine refrigerators) should be double or triple-pane models, all appliances should be enclosed (including display fridges), the insulation surrounding walk-in refrigerators and freezers should be continuous (on all six faces), and a set of double airtight and insulated doors should be employed for access to the walk-in refrigeration spaces. The result is a significant improvement in indoor conditions, which was quickly noticed and praised by the kitchen staff at both universities, soon after the kitchens went into operation.
Eliminate island stations
By their nature, hoods that are positioned over island stations are going to be less efficient and demand more airflow than wall-mounted hoods. Sanchez notes that a proposed island station for pasta in the Harmony Commons kitchen would have required 1,472 cfm of exhaust. Kitchen ventilation design requirements showed that a similar station, when situated against a wall, would only require 800 cfm of exhaust. That’s a reduction of 46%!
Shielding and positioning the hoods
In keeping with this reasoning, Sanchez found that shielding further exhaust hoods on three sides and adjusting their height to make them closer to the cooking surface can further optimize extraction rates. Literature research showed that cutting the distance between the surface of an electric stove and the exhaust hood from 1.6m to 1.2m resulted in a 22% decline in extraction rate, from 796 m3/h to 620 m3/h.
Centralize high exhaust stations
How appliances are organized beneath exhaust hoods is also important. Sanchez notes that when there are several pieces of kitchen equipment grouped in working stations, the equipment with the highest ventilation demand should be placed in the center of that station (and associated exhaust hood). Equipment with more moderate demands can then be placed further away from the center. The least demanding equipment can be located on the periphery. Careful placement ensures that the hood does not have to work as hard to maintain good indoor conditions, which means less intake air that needs to be introduced and conditioned.
System Improvements
Until this point, the tips have been mostly cost-neutral or involved minimal increases in upfront costs, relying on best practices on kitchen layout design. Systems upgrades are different. Though they can increase upfront costs, these more sophisticated components can allow design teams to further enhance overall system efficiency and reduce operational costs.
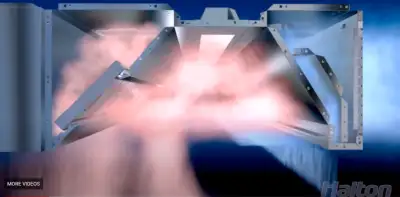
High-capture efficiency hoods
High-capture efficiency hoods use proprietary technologies to improve the capture of thermal plumes and pollutants without increasing the extract ventilation rate. In both kitchens, Sanchez says that the team used hoods engineered by Halton that use two sets of nozzles to introduce a flow of fresh air at the front of the hood and at its sides (see Figure 2). This effectively creates a curtain of air that helps to contain the plume emitted by the cooking equipment, directing it toward the exhaust hood and reducing the exhaust flow rate requirements (and in turn the required flow rate of conditioned make-up air in the kitchen).
Splitting air
As the fresh air introduced by the aforementioned Halton system is designed to contain and direct thermal plumes and smoke emitted from kitchen equipment back into the exhaust stream, it does not need to be conditioned. Consequently, this dedicated hood air curtain can be brought in directly from outside, which reduces energy costs without sacrificing air quality for kitchen staff or other occupants.
Demand control ventilation
Demand control ventilation systems use a combination of sensors that can allow the system to run well below capacity (in some cases averaging between 65% and 70% of the max flow rates) even during periods of high use, right sizing the exhaust flow rates to the kitchen needs rather than over-sizing them. Temperature sensors are installed through the kitchen area to monitor conditions in real time. These sensors measure ambient temperatures within the kitchen and in ductwork, while infrared sensors are trained on the surface areas of cooking equipment, allowing for extremely responsive, on-demand ventilation.
These systems are also “smart” in the sense that they can anticipate spikes in demand. For example, the system will switch on and provide moderate ventilation when it senses a surface temperature getting hot. However, when the surface gets extremely hot, and then becomes ice cold, the system will quickly spring into high gear.
While this seems paradoxical, Sanchez says that this is a learned behavior. The system recognizes that frozen food has just been introduced to a very hot pan. “This is the exact moment where you need the most ventilation,” she says.
“What is the goal of demand control ventilation?” Sanchez asks. “To ventilate only when you need it, as much as you need it.”
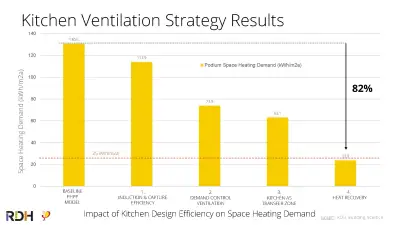
The Results to Performance
According to the PHPP modeling (see Figure 3), the space heating demand for the non-residential portion of Cheko’nien House was 41.4 kBtu/ft2/yr (130.6 kWh/m2/yr) at baseline. The use of more efficient kitchen appliances and improvements in design brought the figure down to 36.1kBtu/ft2/yr (113.9 kWh/m2/yr). Demand control ventilation brought it down further, to 23.4 kBtu/ft2/yr (73.9 kWh/m2/yr). Finally, the use of a cascading approach to the kitchen supply air (not detailed in this article) got the figure to 20.0 kBtu/ft2/yr (63.1 kWh/m2/yr).
While this represents more than a 50% decline in space heating demand, it was still more than double the 8 kBtu/ft2/yr (25 kWh/m2/yr) target the team had set for the non-residential zone. To bring the figure down below 8 kBtu/ft2/yr (25 kWh/m2/yr), they would need to introduce heat recovery on the ventilation system.
Heat Recovery
Whereas design improvements and systems improvements represent upgrades to either industry-standard strategies or equipment, introducing heat recovery systems like heat recovery ventilation (HRV) to the equation improves total efficiency but also constitutes a new upfront cost, due to the departure from the default direct exhaust ventilation approach. The efficiency of the heat recovery systems used in kitchen ventilation systems is also greatly reduced from a standard multi-residential heat recovery ventilation system. Due to the presence of excessive moisture, grease, and other pollutants, simpler heat recovery systems—with easier maintenance access to reduce fire hazards—are used, and able to achieve heat recovery in the 40-60% range. This energy efficiency measure still can account for significant reductions in space heating demand.
Even when operating well below its maximum capacity, the HRV system installed at Cheko’nien House brought the space heating demand down from 20.0 kBtu/ft2/yr (63.1 kWh/m2/yr) to 7.6 kBtu/ft2/yr (23.8 kWh/m2/yr), representing a decline of 82% from baseline. It also brought the team below their target figure of 8 kBtu/ft2/yr (25 kWh/m2/yr) for the non-residential zone, thus paving the way for PHI certification for the entire building, which they were awarded in June 2024.
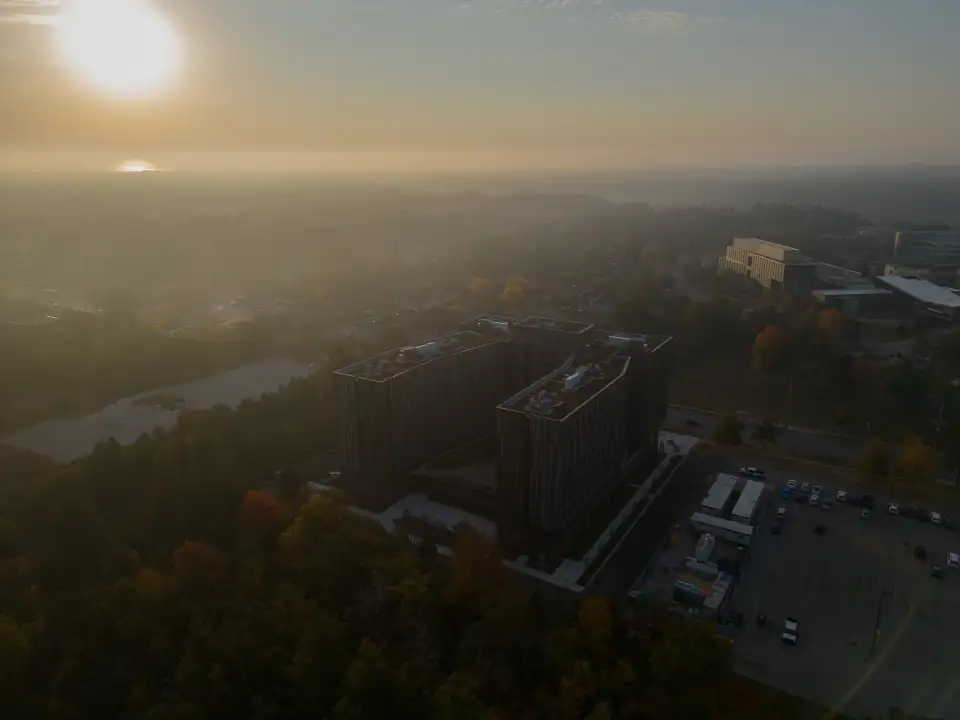
Final Thoughts for Designers
This is most certainly not a comprehensive review of every efficiency strategy for commercial kitchens, nor does it replace the in-depth technical review specific to each project. Moreover, it does not consider the fine tuning that can only result from an examination of the real-world data, which Sanchez hopes to review for both Harmony Commons and Cheko’nien House in 2025.
However, what does seem clear is that dramatic improvements in energy efficiency and greenhouse gas emissions can be achieved by making design and systems upgrades that don’t demand a significant increase in upfront costs. Many of these upgrades simply require better planning, understanding, and coordination to optimize kitchen layouts and ventilation systems. And as per any high-performance, low-carbon buildings, the experience acquired on these projects also shows that delivering a commercial kitchen to meet Passive House levels of performance is, as Sanchez describes it, a team sport, which starts from the very project outset.
Learn More About Commercial Kitchens
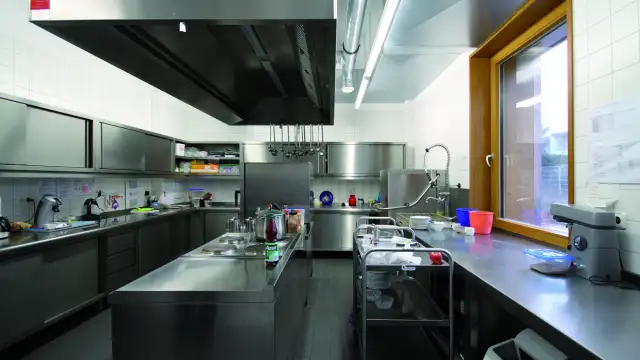
Commercial Kitchens in Passive House Construction: Insights from Magdalena Patyna of the Passive House Institute
Patyna shares some insights about the challenges and solutions that arise when designing a commercial kitchen for a Passive House project.
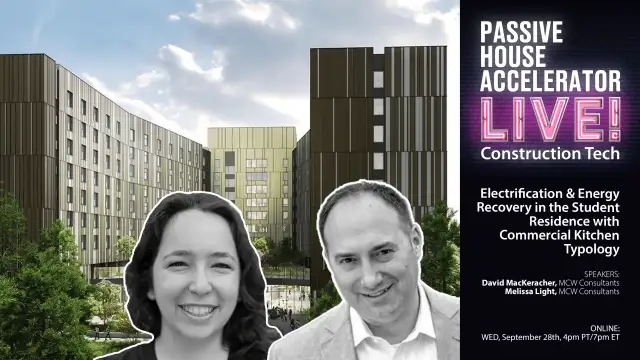
Electrification & Energy Recovery in Student Residence: Commercial Kitchen Typology
David MacKeracher and Melissa Light of MCW Group share how they integrated a full commercial kitchen into U of T’s all-electric, Passive House-certified student residence.
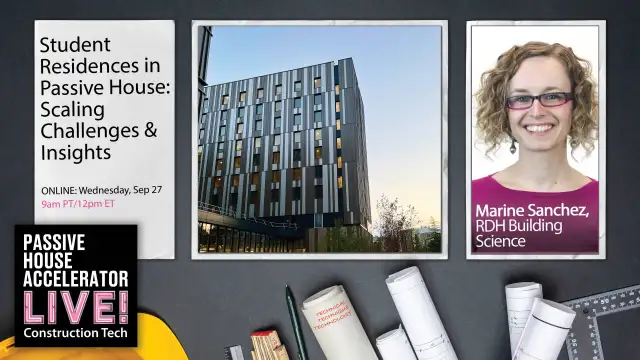
Student Residences in Passive House: Scaling Challenges & Insights
Marine Sanchez talks about the challenges and successes of the construction of the first generation of PH student residences in North-America.